Understanding solar PV arrays
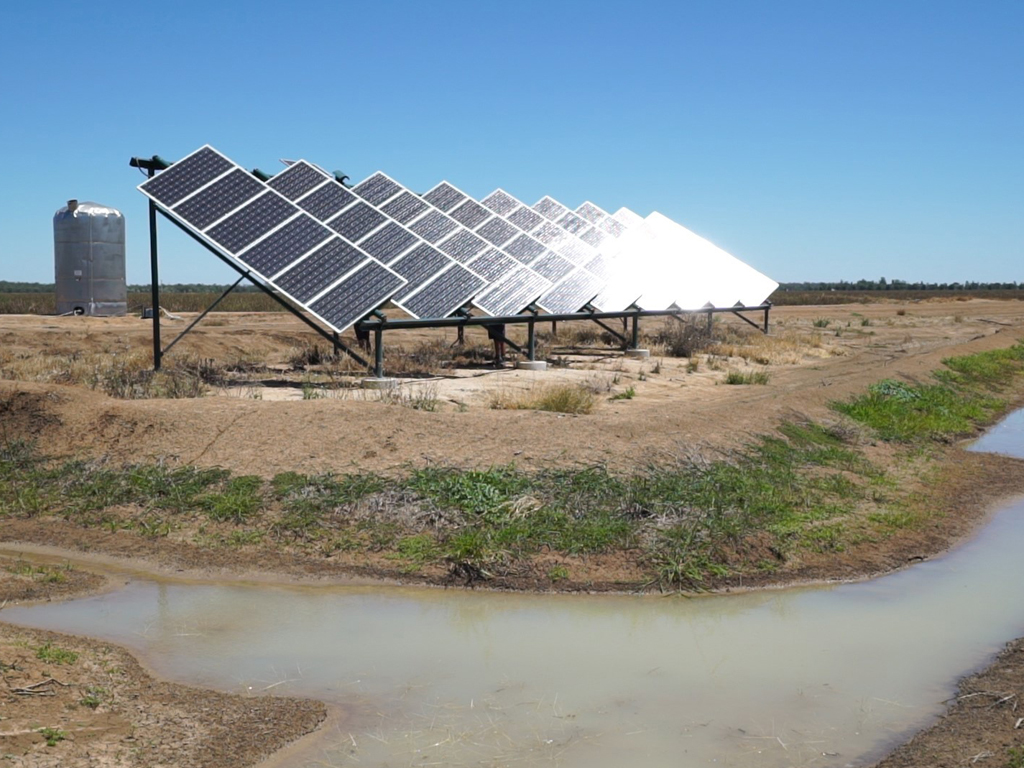
A solar photovoltaic (PV) array is an electrical configuration of solar modules that produce DC electricity when exposed to solar radiation. The solar engineer or installer will consider the following factors when designing an array to meet the electricity needs of your pumps:
- Electrical characteristics: the I-V curve and the maximum power point;
- Operating conditions: the effects of solar radiation levels and temperature;
- Configuring an array: connecting modules in series and in parallel;
- Datasheet values: ratings of a PV module;
- Efficiency and power;
- Composition and types of solar modules;
- Solar array positioning: taking into account the movement of the sun;
- Array positioning: shading;
- Solar array output: system yield according to solar resource; and
- Mounting systems.
Electrical characteristics: I-V curve and maximum power point
Solar modules contain photovoltaic (PV) cells that convert energy from the sun into electrical energy. These cells produce DC electricity that can be used either by AC-compatible appliances such as many household electrical items, or by an AC water pump.
The electricity produced by a PV module is characterised by the I-V curve: this depicts the relationship between the current (I) and the voltage (V) of the cell (Fig. 11 a). For a given set of operating conditions, the PV module can be manipulated at any point along the I-V curve. For every operating voltage, as shown on the x-axis, there is a corresponding current output, as shown on the y-axis.
One point along the curve will produce the most power. This point is known as the maximum power point (MPP) and is located at the ‘knee’ of the curve (Figure 11 b). Operating a PV module at its maximum power point will result in the greatest possible amount of power. Sometimes, however, it is more beneficial to operate the PV module at a specific voltage or current value that does not correspond to the maximum power point. A maximum power point tracker (MPPT) is the most common way to control the electrical output of a PV module.
a) The I-V curve of a PV module (current is typically abbreviated as ‘I’ although its units of measure are Amps); b) the maximum power point of the I-V curve is at the red dot. Note: This point corresponds to the maximum point on the blue ‘power output’ curve.
Key notes |
|
Operating conditions: the effect on solar PV pumping of solar radiation levels and temperature
The operating conditions – namely, the solar radiation levels and temperature of the module, affect the I-V curve of a module (Fig. 12 a). In essence, as solar radiation (technical name: ‘irradiance’) levels increase, more current is available; hence, there is more available power. As cell temperature increases, less voltage and hence less power is available (Fig. 12 b).
Figure 12: a) Module power output increases with levels of solar radiation (irradiance); b) module power output decreases with the temperature of the module.
Floating arrays: can these increase output? |
The ideal operating conditions for a solar array are high levels of solar radiation and cool temperatures. Days with high solar radiation are usually hot, which inevitably means the array will heat up. Floating solar arrays are gaining popularity. The array can float above, or sit just below, the surface of the water. The water underneath helps cool the modules, resulting in higher power output. The example shown below showed a 40% improvement in efficiency and reduced evaporation by 1-3m pa. The additional advantage of reducing evaporation is especially useful. |
![]() Figure 13: A floating solar array in a dam (Courtesy of HydroSun). See http://bit.ly/1CTuv09 or wsaa.asn.au for more information.
|
The amount of solar radiation at a site varies throughout the day. Typically, lower levels of solar radiation are emitted in the mornings and afternoons, and higher levels occur in the middle of the day. The total daily solar radiation received at a site also varies throughout the year. Daily solar radiation levels are greater in the summer when there are more daylight hours and higher solar radiation levels throughout the day (Fig. 14). Cloud cover will reduce the amount of solar radiation reaching the array, but they will continue to generate some power even on cloudy or overcast days.
Figure 14: Difference in solar radiation levels across the day between summer and winter for Wagga Wagga (data based on the Australian Solar Radiation Data Handbook, www.exemplary.com.au).
Note regarding units of measurement |
Instantaneous solar radiation power received at a site is measured in kW/m. The amount of solar radiation energy received in an hour at a site is measured in kWh/m. The amount of solar radiation energy received in a day or more is measured in kWh/m. or in peak sun hours (PSH), where 1 kWh/m. is equivalent to 1 PSH. Find more information on units of measurement in Appendix B. |
Key notes |
|
Configuring an array: connecting modules in series and in parallel
A solar array is the electrical combination of a number of solar modules. The power output of this array will be the sum of the power outputs of the combined solar modules.
The method by which the solar modules are configured will determine the electrical output characteristics (namely, current and voltage) of the solar array. Modules can be connected in series (Fig. 15 a), in parallel (Fig. 15 b), or in a combination of the two.
Simply put, (i) connecting modules in series means that the voltages of the modules are summed but the current output stays the same, and (ii) connecting modules in parallel means that the currents of the modules are summed but the voltage output stays the same.
Figure 15: a) modules connected in series; b) modules connected in parallel.
The implications of this are that two arrays can have the same power output but will operate at different current and voltage outputs according to their configuration. Simply matching an array’s rated power output to the power requirements of the pump or motor is not enough: when designing a solar PV array, you must ensure that the array’s voltage and current outputs match the pump’s requirements.
Key notes |
|
Datasheet values: ratings of a PV module
As explained in Section 1: opportunities for solar powered Pumping, the output of a PV module is affected by the amount of sunlight it receives and the daytime temperature. The manufacturer will stated output of a PV module is calculated using a standard set of operating conditions known as Standard Test Conditions (STC). These conditions are as follows:
- solar radiation levels (irradiance) = 1,000 W/m.,
- cell temperature = 25 C, and
- air mass (a unit of air volume) = 1.
As all modules are tested under these conditions, different modules can be compared using their ‘rated’ outputs – that is, their outputs as calculated under STC. Standard Test Conditions do not necessarily represent ‘real-world’ conditions; the STC solar radiation level is higher than that normally experienced and the STC cell temperature is very low (‘cell’ temperature is not the ambient temperature; it represents the operating temperature of the module’s silicon cells). Therefore, modules should not be expected to produce their rated power in all instances.
Key notes: |
|
Efficiency and power
The efficiency value given in a solar PV module’s datasheet relates to the relationship between the amount of sunlight received (W/m.) and the amount of power generated (W) by that module. So a module’s efficiency value relates to the size (i.e. surface area) of the module that is needed to produce a certain amount of power (Fig. 16). It does not mean that a lower-efficiency module will produce less power than a higher-efficiency one.
Figure 16: When two modules have the same power output, the more ‘efficient’ module will take up less space.
High-efficiency modules are usually pricier and are best suited to applications where installation space is limited.
Example |
A farmer is going to install a 1kW solar PV array and has a choice between two brands of module. Both modules are approximately the same physical size.
|
Array configuration |
Power output The power output of the array will be 1kW under standard test conditions, regardless of which modules are chosen. The difference between the arrays will be that an array comprising the low-efficiency modules will need an additional module to achieve a 1kW output under STC, and will hence take up a greater surface area. |
Cost |
Key notes |
|
Composition and types of solar modules
Photovoltaic modules are made up of PV cells electrically connected and sandwiched between a backing sheet and a glass front, with transparent glue holding it all together. Most modules have an aluminium frame that provides mechanical protection for the modules. Three main types of solar modules are available commercially: monocrystalline, polycrystalline and thin-film.
Monocrystalline modules
Monocrystalline cells (Fig. 17) are dark blue in colour and almost square, missing corners due to the method of production. They are produced from a single cylindrical crystal of silicon cut into thin circular wafers. To increase the cell density in a module, four edges are then cut from each of the round cells, allowing them to be packed closely.
Monocrystalline modules are typically more efficient than other types of solar modules, with commercial efficiency levels of around 18 to 21 percent. They also tend to have higher cost-to-power ratios than other solar modules.
Figure 17: A monocrystalline module.
Polycrystalline modules
Polycrystalline (or multicrystalline) cells (Fig.18) are usually dark or light blue in colour and are easily identifiable by their distinctive grain structure that glitters in sunlight. They are created by allowing molten silicon to cool in a block, forming various crystal structures in the process, then slicing the block into thin wafers that are processed into PV cells.
Typically, polycrystalline modules are less efficient than monocrystalline ones, with commercial efficiency levels of around 15 to 18 percent; however, they also tend to have lower cost-to-power ratios than monocrystalline modules.
Figure 18: A polycrystalline module.
Thin-film modules
Thin-film modules (Fig. 19) can vary a lot in appearance. Commonly, they have a smooth, glassy finish and no cell division lines. They are made by applying a photoactive semiconductor in thin layers to a substrate that may be glass, metal or plastic.
Thin-film modules are typically the least efficient type of solar module; however, they have lower production costs and function well in low light conditions. They are less affected by high operating temperatures, which can make them well suited to very hot climates.
Figure 19: A thin-film module.
Key notes |
|
Solar array positioning: taking into account the movement of the sun
A solar module will receive the most amount of solar radiation – and therefore produce the greatest amount of power – if it faces directly into the sun; however, the sun moves throughout the day and the year.
- Daily movement: Each day, the sun moves from the east, where it rises, to the west, where it sets. In New South Wales, the sun will nearly always be in the northern part of the sky except in the morning and evening hours of the summer months. The compass position of the sun is known as its ‘azimuth’ and is measured in degrees.
- Yearly movement: In the summer months (Fig. 20 a), the sun rises approximately in the south-east of the sky and sets in the south-west. Shortly after rising, it moves into the northern part of the sky, and in the middle of the day it is very high in the sky. In the winter months (Fig. 20 b), the sun rises approximately in the north-east of the sky and sets in the north-west, staying in the northern part of the sky, and is lower in the sky in the middle of the day than it is in the summer.
Figure 20: a) In summer, days are longer and the sun is higher in the sky at solar noon; b) in winter, days are shorter and the sun is lower at solar noon. In both pictures, the sun stays in the northern part of the sky for most of the day.
There are three main options for maximising the amount of direct solar radiation received by a solar module:
- fixing the array in the optimal tilt and orientation;
- using a manual tilting frame; and
- using a single- or dual-axis tracker that follows the sun.
Fixed array
Fixing the array so it has the optimal tilt and orientation is generally the cheapest option and the one that involves the least maintenance. The greatest possible annual power output from the array will be obtained if the array is orientated to the north and tilted at an angle equal to that of the site’s latitude. However, it may be better to orient and tilt the array so that it generates more power at times that match your particular pumping regime, even if it will generate less power over the year.
The latitude of Tamworth, for example, is 31⁰ S, so tilting the array at 31⁰ would give the highest annual solar radiation and hence, the highest annual power output at this location (as shown in Table 1).
If the array is installed so that it sits at a higher elevated tilt (i.e. at a steeper angle), it will have greater exposure to sun in winter, when the sun traces a lower arc across the sky. A steeply tilted array will receive more direct solar radiation and hence will produce more power in winter. During the summer months, however, such an array will receive less direct solar radiation and will produce less power than its potential maximum (as shown in Table 1 and Fig. 21 as ‘Tilted at 46⁰’).
Installing a solar PV array that gives preference to the sun’s trajectory over winter could suit farmers whose water pumping requirements are higher in winter or are year-round.
If an array is installed so that it sits at a lower-than-optimal (flatter) tilt angle, it will receive less direct solar radiation and produce less power over the winter months but in summer, flatter arrays will receive more direct solar radiation and produce more power than their steeper equivalents (shown in Table 1 and Fig. 21 as ‘Tilted at 16⁰). An array installed to preference the summer-sun could be suitable if your pumping requirements increased over summer.
An explanation of how to calculate levels of solar radiation received by arrays at different tilts is given in Section 8.2.
Table 1: Average daily solar radiation in Tamworth, latitude 31⁰ S, at different tilts. Maximum values for summer and winter, and average annual values, in red (Source: NASA Surface Meteorology and Solar Energy website).
Figure 21: Tilting the modules at an angle less than the site’s latitude (16⁰) means they will receive more solar radiation in summer and less in winter. Tilting them at an angle greater than the site’s latitude (46⁰) means they’ll receive more solar radiation in winter, less in summer.
Minimum tilt for self-cleaning = 10 degrees. |
It is recommended that solar modules be installed at a minimum tilt angle of 10⁰ so that any build-up of dirt on the modules will be washed off naturally by rain and what remains can be removed easily with a bucket of water. Without this self-cleaning, modules accumulate dirt and dust that reduces their power output. |
The optimal orientation for a solar PV array in the Southern Hemisphere is facing north. This orientation will result in the greatest possible generation of power throughout the day. Alternatively, modules could be positioned to face east or west so as to maximise morning or afternoon power generation. This orientation might be suitable for a pumping application that requires water to be pumped directly to it (such as drip irrigation) and for situations requiring more water in the morning (in which case, the array should be east-facing) or in the afternoon (in which case a west-facing array may be preferable).
For applications incorporating water storage, it is usually better to angle solar PV modules to the north to maximise solar generation, and so your water storage can be the water-delivery source in the mornings and/or afternoons.
Manual tilt
Using a frame that can adjust the tilt of the array is a cost-effective option if you’re seeking to maximise the amount of power generated by an array. The array is tilted manually at the start of each month or season so that it receives more direct solar radiation for that period. This option requires routine servicing of its moving parts.
The optimal tilt angle for each month/set of months is calculated according to changes in the altitude of the sun over the months. Four key times of year can be used to determine this:
- Every year, there are two equinoxes (days when the sun is directly above the equator), occurring in the months of March and September. In these months, the optimal array tilt is equal to the latitude of the site.
- The winter solstice (shortest day of the year) occurs in June. In this month, the optimal tilt for a solar array is approximately 23⁰ higher (steeper) than it is during an equinox; i.e. optimal tilt = latitude of the site + 23⁰.
- The summer solstice (longest day of the year) occurs in December. During this month, the optimal array tilt is approximately 23⁰ lower (flatter) than it will be during an equinox; i.e. optimal tilt = site latitude – 23⁰.
Table 2: Monthly and quarterly manual tilt plan.
Tracking systems
A tracking system moves the array so that it directly faces the sun as much as possible. Installing a tracking system along with a solar PV array is a more expensive option that should result in greater generation of power, especially in the mornings and afternoons.
There are two main forms of trackers:
- Single-axis trackers: These rotate the array in the east-west axis only, following the sun at a fixed angle of elevation from the time it rises in the east until it sets in the west (Fig. 22). Installing a single-axis tracker for your solar PV array results in higher power output in the mornings and evenings (Fig. 23).
- Dual-axis trackers: These rotate the array on an east-west axis and tilt it on a second axis so that it is angled directly towards the sun at all parts of the day.
Figure 22: The single-axis tracker follows the sun from east to west.
Figure 23: A single-axis tracker will result in higher power output from a solar PV array in the early mornings and evenings. Cooler morning temperatures mean that the array’s morning output will be slightly higher than its evening output; a module’s power output reduces as it heats up.
The main advantage of using a tracker with a solar-powered pump is that it enables the pump to generate sufficient power to operate earlier in the mornings and later in the afternoons: for this, the pumping system uses a solar controller (for more information on solar controllers, go to Section 2: System controllers).
The main disadvantage of installing a tracker is that it will need to be checked and serviced regularly. Without regular maintenance the tracker may break down, leaving the array stationary and operating at low efficiency. Also, tracker systems may be more vulnerable to damage by high winds than fixed arrays.
An alternative to installing a tracking system is to install a larger static solar array, or an array made up of modules facing both east and west to capture morning and afternoon sun. Lack of space is rarely an issue for rural solar arrays and the incremental cost of adding modules is relatively small, so usually this is a more cost-effective option.
Example |
To illustrate the differences in output between a fixed tilt system, a manual tilt system, a single-axis tracker and a dual-axis tracker, the average daily solar output for each month has been calculated for Sydney, NSW (Table 3 and Fig. 24). Data was sourced from the PVWatts v.1 calculator supplied by the National Renewable Energy Laboratory (NREL) (rredc.nrel.gov/solar/calculators/pvwatts/version1). |
The benefit derived from increased solar array output when using a tracker should be weighed against the increased equipment and maintenance costs of having to install a more complex tracking system. It could be more economical to install a larger fixed array than a manual tilt or tracker. |
![]() Table 3: Monthly comparison between different mounting options.
|
![]() Figure 24: Average daily solar radiation received each month for different mounting options.
|
Key notes |
|
Array positioning: shading
If the solar array is shaded in any way, its power output will be reduced. If there is continuous shading at the same position on the solar modules, the modules can develop ‘hot spots’ that can damage them permanently. Incidental shading is not static, as it moves throughout the day and year, following the movement of the sun. It is important that a comprehensive analysis is undertaken to pinpoint possible sources of shading and to confirm where shading will impact the modules at different times of the day and year.
Possible sources of shading include:
- vegetation – trees, bushes, long grass, leaves;
- structures – buildings, shelters, fences, telegraph poles;
- landforms – hills, rocks; and
- the array itself – not leaving enough room between rows of tilted modules can cause one row to shade the row behind it.
There are professional shading analysis tools available that enable you to determine shading accurately across a full year. Examples of these tools include Solar Pathfinder and Solmetric Suneye.
Table 4 provides an estimate of the shadow that would be imposed by an object a metre high at six different latitudes in NSW in June (as shadows are the longest in winter).
Key notes |
|
Figure 25: As shown in Table 4, the shadow of a metre-tall tree in Lismore at 1pm on a June day would be 1.4m long and its direction would be 1.3m south and 0.5m east.
Table 4: Shadow length of a 1m object for various cities in NSW on an average June day.
This table gives two key parameters for the shadow of a metre-tall object: the length of the shadow and its direction (as shown in Fig. 24). For a taller or shorter object, multiply these values by the height of the object in metres.
Solar array output (system yield) according to solar resource
The amount of power generated by a solar array is directly proportional to the solar resource available throughout the day. Two key calculations can be performed to determine the system yield:
Power output (instantaneous output)
- Power output = instantaneous solar radiation (kW/m.) x rated array power (kW) x system efficiency
Energy output (output over time)
- Hourly energy output = hourly solar radiation (kWh/m.) x rated array power (kW) x system efficiency
- Daily energy output = daily solar radiation (kWh/m. or PSH) x rated array power (kW) x system efficiency
Note: The total array power is equal to the sum of all the modules’ power, as shown in the datasheet.
System efficiency relates to factors that reduce the power/energy output of the modules. Several factors can affect system efficiency; they include:
- temperature – a higher cell temperature will reduce the power output;
- shading – any shading will reduce the power output;
- dirt – any soiling of the modules will reduce the power output;
- cable losses – power is lost as electricity runs through cables; and
- orientation and tilt losses – the orientation and tilt of the modules affect the amount of solar radiation that hits the modules, in turn affecting the power output.
Is system efficiency different to module efficiency? |
System efficiency relates to losses in the equipment’s operation. This is different to PV module efficiency, which relates to how well a solar PV module converts solar radiation into electricity. The module’s efficiency is already taken into account in the array’s rated power. |
Example 1 |
A 1.2kW array has been installed to run a bore pump. The measured sunlight intensity is 0.76kW/m. and the system efficiency has been calculated to be 70%. The power output of the array is: |
1.2kW x 0.76kW/m. . 0.7 = 0.638kW |
Example 2 |
The 1.2kW system installed to run a bore pump is exposed to 5.28kWh/m. over the course of one day, with a system efficiency of 70%. The energy output of the array over the day is: |
1.2kW x 5.38kWh/m. . 0.7 = 4.52kWh |
For some low-volume solar pumping installations, the solar array is intended to drive a DC pump directly. A pump motor will have an initial start-up power requirement that is greater than the motor’s running power. In such an installation, the power output of the array must match the start-up power required. If the solar PV array cannot supply that initial power requirement, the pump will not start even if the array has enough power to run the motor once started.
Key notes |
|
Mounting systems
Solar arrays can be installed on structures (roof-mounted), on the ground, on poles or on trackers. The suitability of various mounting options is summarised in Table 5.
Roof Mounted
|
A roof-mounted array can be installed on a suitable existing structure (such as a shed). This is the standard mounting option for urban grid-connected solar PV systems, so there are many competitively priced products available. The size of the array that’s possible is limited by the available installation area on the roof. |
Ground Mounted
|
A ground-mounted array can be positioned for best exposure to sunlight. However, this option requires a suitable area of ground area and type of soil. Many installation methods and products (eg. pile-driven, concrete foundations) are available to suit different scenarios. This option may require extra maintenance to prevent vegetation from growing up and shading the modules and requires protection from stock. |
Pole Mounted
|
A potential installation method for solar PV power in areas where ground- or roof-mounting is not suitable is to mount the array on poles; however, available products will limit the maximum number of solar modules that can be pole-mounted, and there are relatively few products available for this type of installation. Installing a pole-mounted solar PV array is a more detailed operation than other forms of mounting, and the structural loading on a pole-mounted array must be determined in advance. |
Floating system
|
A floating or fixed system on top of a body of water can offer multiple benefits. The array can help to slow evaporation of valuable reservoir water by covering it. The solar cells on the array will also remain cooler and therefore operate more efficiently as heat is more easily absorbed by the surrounding water. Most importantly, a floating system will not require relinquishing valuable farmland. This can be particularly beneficial for horticultural or other farms with high-value produce.
|
Tracking system
|
Tracking devices enable a solar array to ‘track’ the sun through the sky, optimising the solar contribution to the pumping system. Tracking systems are more expensive than any of the ‘fixed’ options and while trackers can boost output, they may also introduce a point of failure for the pumping system because of the tracker’s moving parts and ongoing maintenance requirements. |
A solar PV array could also be installed on a mobile structure, such as a trailer, for a smaller solar pumping system that can be moved around. Care must be taken that the structure can support the array and that it is suitably earthed to prevent the risk of electrocution.
In all options, the mounting structure must be strong enough to withstand the forces of wind on the array, known as wind loading. Australian standard AS 1170.2 covers wind loading of a structure and the array-mounting structure you choose should adhere to this standard.
Key notes |
|